1.1 Deformation of hardened concrete moduli of elasticity
Deformation of hardened concrete:
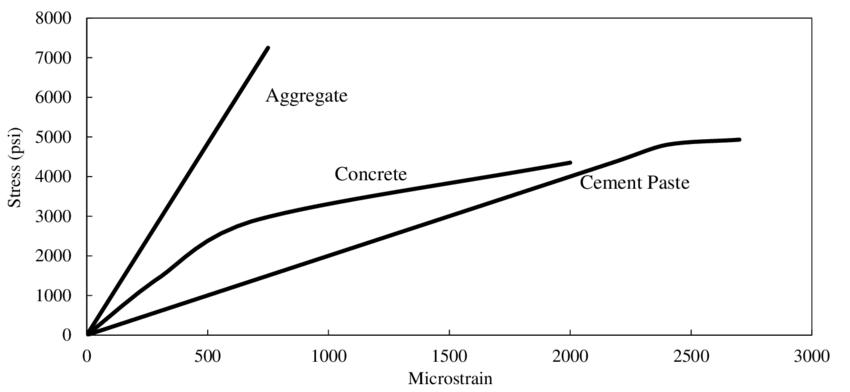
Following strains are responsible for the deformation of concrete:
a. Elastic strain:
- Instantaneous deformation that occurs when an external stress is first applied.
b. Shrinkage strain:
- Deformation that occurs due to the loss of moisture from concrete.
c. Creep strain:
- Deformation that occurs in the direction of force applied.
d. Thermal strain:
- Deformation due to change in temperature.
Modulus of elasticity:
- Defined as the slope of the relationship between stress and strain.
- It is a measure of stiffness or resistance to deformation of a material.
- Higher modulus indicates stiffer concrete with lesser deformation.
Types of modulus of elasticity:
1. Static modulus of elasticity:
- It is the value of modulus of elasticity determined by actual loading of concrete.
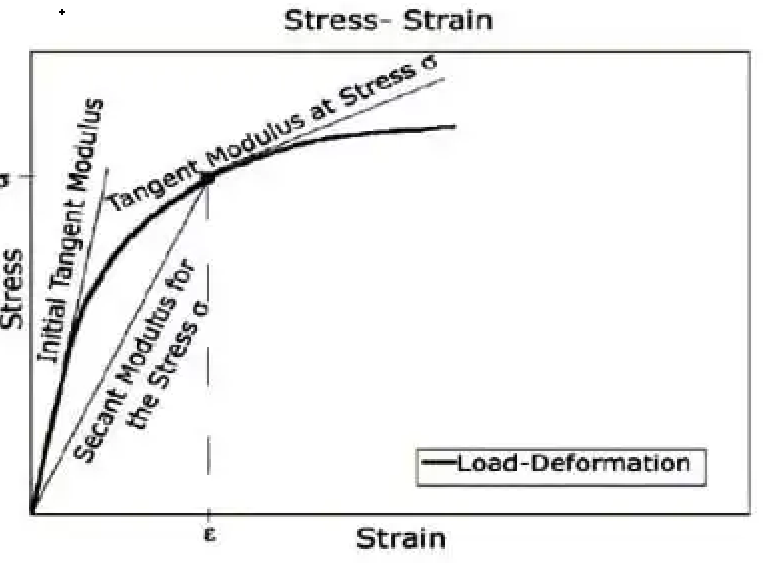
Types of static modulus of elasticity:
a. Initial tangent modulus:
- Given by the slope of a line drawn tangent to the stress- strain curve at the origin.
b. Tangent modulus:
- Given by the slope of a line drawn tangent to the stress – strain curve at the origin.
c. Secant modulus:
- Given by the slope of a secant joining origin and a fixed point on curve.
d. Chord modulus:
- Given by the slope of a line drawn between two point on the stress strain curve.
2. Dynamic modulus of elasticity:
- Elasticity of concrete under dynamic loads such as longitudinal and flexural vibration.
3. Flexural modulus of elasticity:
- Ability of a material to bend.
1.2 Creep, shrinkage and thermal expansion
Creep:
- Defined as gradual increase in strain (deformation) under sustained stress (or loads).
- Dependent on quality of cement paste and age of concrete.
- The amount of creep is expressed in terms of creep coefficient.
Creep coefficient (Cc) = Creep strain / Elastic strain
Factors affecting creep:
- Higher w/c ratio higher the creep.
- The stiffer the aggregate the smaller the creep.
- More aggregate fraction less is the creep.
- Increase in temperature leads to increase in creep.
Shrinkage:
- Reduction in the volume of a freshly hardened concrete under the condition of ambient temperature and humidity.
- It is caused by loss of water through evaporation or by hydration of cement.
Types:
1. Plastic shrinkage:
- Occurs due to the loss of water by evaporation from freshly prepared concrete while cement paste is plastic.
- At larger cement content of mix, plastic shrinkage of concrete is higher.
2. Drying shrinkage:
- Occurs due to the loss of water by evaporation from freshly hardened concrete exposed to air.
3. Autogenous shrinkage:
- Occurs due to loss of water by self – desiccation of concrete during hydration.
4. Carbonate shrinkage:
- Occurs when the concrete is exposed to air containing carbon dioxide.
5. Thermal shrinkage:
- Occurs due to excessive fall in temperature.
Factors affecting shrinkage:
- Higher the w/c ratio larger the shrinkage.
- Low relative humidity more the shrinkage.
- The lighter the aggregate more the shrinkage.
Thermal expansion:
- Concrete expands on increasing the temperature.
- The coefficient of thermal expansion depends upon nature of cement, aggregate, relative humidity and size of concrete. In normal condition the expansion mainly depends upon aggregate.
1.3 Fatigue, impact and cyclic loading
Fatigue:
- Failure of a material by fracture when it is subjected to a cyclic stress.
- Fatigue is the result of repeated application of stress to the concrete.
Two types of failure in fatigue takes place in concrete are:
a. Failure occurs in sustained load (slowly increasing load)
b. Failure occurs under cyclic or repeated loading.
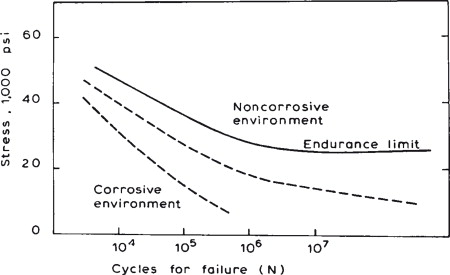
- For constant load of alternating stress, the fatigue strength decreases as the number of cycle increases.
Log N = (1 – Fmax/Fc)/ β(1 – Fmin/Fc)
Where,
Fmax = Maximum stress in a cycle
Fmin = Minimum stress in a cycle
Fc = Strength under static loading
Β = 0.085
Impact and cyclic loading:
- The load that is applied suddenly at short time interval is called impact load. The strength that shows impact load is called impact strength.
- Impact strength increases with:
a. Increase in rate of loading.
b. Increase in tensile strength.
c. Decrease in aggregate strength.
- The CEB – FIP mode code (1990) recommends following relation for impact strength.
Fc,imp = fc * (σs / σo) α
where,
Fc,imp = Impact compressive strength
fc = Compressive strength of concrete
σs = Impact strength rate
σo = 1 MPa
α = 1 / [5 + 9 * (fc / fco)]
1.4 Effect of porosity, water – cement ratio and aggregate size
Effect of porosity:
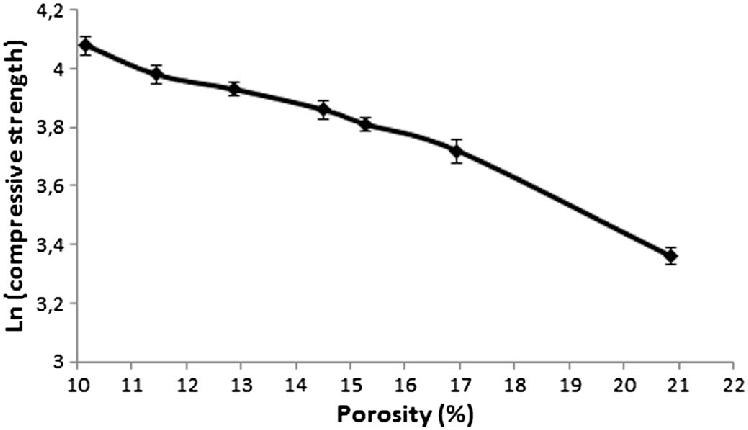
- Primary factor that governs the strength of concrete.
- Strength of concrete decreases with the increase in porosity.
- Permeability of concrete increase with increase in porosity of concrete.
- Factors like w/c ratio, degree of hydration, air content, mineral admixture, aggregates affects concrete porosity.
- Increase in w/c ratio increase the porosity.
Effect of water content cement ratio:
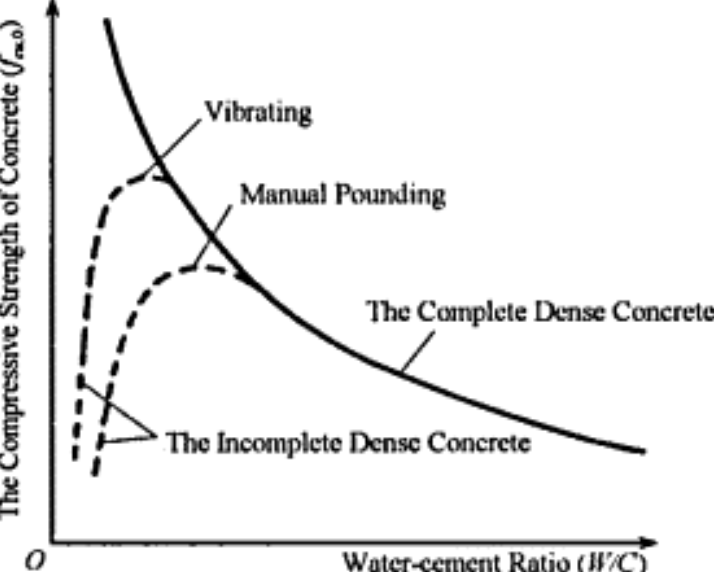
- W/C ratio is an index of strength of concrete. The strength of cement paste increases with increase in cement concrete and decrease in water content and air content.
- High w/c ratio increase the porosity and permeability of concrete and reduce its durability.
- From Abram’s law it is found that the cement requires about 1/5 to 1/4 of its weight of water to hydrate completely.
Mathematically,
C = A /Bx
Where,
C = Compressive strength of concrete
A, B = Empirical constant
x = w/c ratio
Effects of aggregate size:
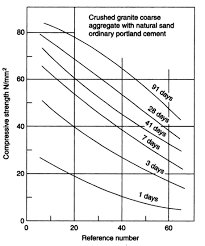
- Aggregate is an important factor affecting the concrete strength. The most important properties of concrete are shape, texture and size of aggregate.
- Large size aggregate reduce specific surface area of aggregate which leads to reduction in bond.
- If constant workability is maintained, strength will increase with cement content.
- High strength or rich mix concrete is adversely affected by the use of large size aggregate. When large aggregate are used due to internal bleeding in transion zone it will become much weak by developing micro cracks with lower compressive strength.
1.5 Durability of concrete
- The ability of concrete to resist weathering action, chemical attack and abrasion while maintaing its desired engineering properties.
Factors that affect durability of concrete:
- W/C ratio
- Compaction
- Permeability
- Temperature
- Aggregate
- Porosity
References:
- Dayaratnam, P. Brick and reinforced brick structure.
- Neville, A.M. Properties of Concrete. England: Pearson Education Limited.
- Hendry, A.W., Sinha, B.P. & Davies, S.R. Design of Masonry Structure. London: E & FN Spon.